# Comparing Magnetic Materials: A Deep Dive into Coercivity, Permeability, and More!
Understanding magnetic materials is crucial in countless applications, from simple refrigerator magnets to advanced medical imaging devices. But with a vast array of options available, how do you choose the right material for your specific needs? This article will explore a range of magnetic materials, comparing them based on key properties like coercivity, permeability, saturation magnetization, and Curie temperature. We’ll break down the jargon, present data in a clear and accessible format, and provide insights into their practical applications. Whether you’re a student, engineer, or simply curious about the fascinating world of magnetism, this comprehensive guide will empower you to make informed decisions.
## What is Coercivity and Why Does it Matter for Magnetic Materials?
Coercivity, often measured in Oersteds (Oe) or Amperes per meter (A/m), is a crucial property of magnetic materials. It represents the intensity of the magnetic field required to reduce the magnetization of a previously magnetized material to zero. A high coercivity indicates a “hard” magnet, resistant to demagnetization, while a low coercivity signifies a “soft” magnet, easily magnetized and demagnetized.
Why is this important? Imagine using a magnet in a noisy, high-temperature environment. A hard magnet, with its high coercivity, will retain its magnetization despite external influences. Soft magnets, on the other hand, are ideal for applications like transformers, where rapid magnetization and demagnetization are essential for efficient energy transfer.
## How Does Permeability Affect the Performance of Magnetic Materials?
Permeability, denoted by the Greek letter μ (mu), describes a material’s ability to support the formation of a magnetic field within itself. It’s essentially a measure of how easily a material becomes magnetized in an applied magnetic field. A high permeability means that the material can concentrate magnetic flux lines, making it excellent for applications like transformer cores and magnetic shielding. Low permeability materials, on the other hand, resist the formation of a magnetic field.
Think of it this way: permeability is like how easily water flows through a pipe. A high-permeability material is like a wide, smooth pipe allowing magnetic flux to flow freely, while a low-permeability material is like a narrow, rough pipe restricting the flow. This property is critical for optimizing the performance of various electromagnetic devices. Relative permeability, μr, commonly used, is the permeability of a material divided by the permeability of free space (μ0).
## What is Saturation Magnetization and Its Significance?
Saturation magnetization (Ms) represents the maximum magnetic moment per unit volume or mass that a material can achieve when subjected to a strong magnetic field. In other words, it’s the point where increasing the applied field no longer leads to a significant increase in magnetization. This property is directly related to the material’s atomic magnetic moments and their alignment.
A high saturation magnetization means the material can store more magnetic energy, making it suitable for applications where strong magnetic fields are required, such as motors, generators, and data storage devices. Materials with low saturation magnetization often find use in applications where precise control over weak magnetic fields is needed.
## Curie Temperature and Its Relationship to Magnetic Material Selection:
The Curie temperature (Tc) is a critical parameter that defines the temperature above which a ferromagnetic or ferrimagnetic material loses its permanent magnetic properties and transitions to a paramagnetic state. Above Tc, thermal energy disrupts the alignment of atomic magnetic moments, causing the material to lose its spontaneous magnetization.
Selecting a magnetic material with a Curie temperature significantly higher than the operating temperature is crucial for ensuring stable magnetic performance. For example, if you’re designing a motor that will operate at 150°C, you’ll need to choose a magnetic material with a Curie temperature well above that, perhaps 300°C or higher.
## Alnico vs. Ferrite: A Comparison of Hard Magnetic Materials
Alnico and ferrite magnets are two common types of hard magnetic materials, each with distinct properties. Alnico, an alloy primarily composed of aluminum, nickel, and cobalt, boasts high Curie temperatures and excellent temperature stability. They also exhibit relatively high remanence (the magnetic field remaining after the magnetizing force is removed). However, they are mechanically brittle and have lower coercivity compared to some other hard magnets.
Ferrite magnets, on the other hand, are ceramic materials made from iron oxide and other elements. They are cheaper to produce than Alnico magnets and possess excellent resistance to demagnetization (high coercivity). However, they have lower saturation magnetization and Curie temperatures compared to Alnico.
In der folgenden Tabelle sind die wichtigsten Unterschiede zusammengefasst:
| Property | Alnico | Ferrite |
|——————-|————-|————-|
| Coercivity | Lower | Higher |
| Saturation Magnetization | Higher | Lower |
| Curie Temperature | Higher | Lower |
| Temperature Stability | Excellent | Good |
| Cost | Higher | Lower |
| Mechanical Strength| Brittle | Brittle |
## Neodymium vs. Samarium Cobalt: Which Rare-Earth Magnet is Right for You?
Neodymium (NdFeB) and Samarium Cobalt (SmCo) magnets both belong to the class of rare-earth magnets, known for their exceptional magnetic strength. Neodymium magnets offer the highest energy product (a measure of magnetic strength) and saturation magnetization, making them ideal for applications requiring powerful magnetic fields. However, they have a lower Curie temperature and are more susceptible to corrosion than SmCo magnets.
Samarium Cobalt magnets, while slightly weaker than Neodymium magnets, exhibit superior temperature stability and corrosion resistance. They can operate at higher temperatures without significant loss of magnetic properties, making them suitable for applications in harsh environments.
Choosing between the two depends on the specific application requirements. If maximum magnetic strength is priority and the operating temperature is within limits, Neodymium magnets are the better choice. If temperature stability and corrosion resistance are paramount, SmCo magnets are more appropriate.
## Comparing Soft Magnetic Materials: Silicon Steel vs. Mu-Metal
Silicon steel and Mu-Metal are two widely used soft magnetic materials, each optimized for different applications. Silicon steel, typically containing a few percent silicon, exhibits high permeability and low core loss, making it ideal for transformer cores and electric motor laminations. The addition of silicon increases the electrical resistivity, reducing eddy current losses.
Mu-Metal, an alloy primarily composed of nickel, iron, copper, and molybdenum, possesses extremely high permeability, even higher than silicon steel. Its strength lies in its ability to effectively shield against external magnetic fields. This makes it indispensable in applications like sensitive electronic instruments, magnetic shielding enclosures, and magnetic resonance imaging (MRI) systems. However, Mu-Metal is more expensive and more sensitive to mechanical stress than silicon steel.
## Amorphous Metals: A Cutting-Edge Option for Soft Magnetic Applications?
Amorphous metals, also known as metallic glasses, are alloys that lack the long-range crystalline order found in conventional metals. This unique atomic structure gives them exceptional soft magnetic properties, including high permeability, low coercivity, and low core loss. These characteristics make them promising candidates for applications like high-frequency transformers, magnetic sensors, and power electronics.
One of the key advantages of amorphous metals is their ability to operate efficiently at high frequencies, reducing energy losses due to hysteresis and eddy currents. However, they can be more expensive to manufacture and may have lower saturation magnetization compared to some crystalline soft magnetic materials.
## How Does Temperature Affect Magnetic Material Performance?
Temperature plays a significant role in the behavior of magnetic materials. As temperature increases, the thermal energy within the material increases, disrupting the alignment of atomic magnetic moments. This can lead to a decrease in saturation magnetization, reduced coercivity, and ultimately, demagnetization. The Curie temperature (Tc) is the point at which ferromagnetic behavior is lost entirely.
For applications operating at elevated temperatures, it’s crucial to select magnetic materials with high Curie temperatures and good temperature stability. Consider SmCo magnets over NdFeB magnets, or Alnico magnets over ferrite magnets in such scenarios.
## What Role Does Magnetic Material Play in Data Storage Technology?
Magnetic materials are foundational to data storage technologies, from traditional hard disk drives (HDDs) to advanced magnetic random-access memory (MRAM). HDDs utilize thin films of magnetic materials to store data as magnetized domains. The direction of magnetization within each domain represents a binary bit (0 or 1). High coercivity materials are essential to maintaining the stored data against thermal fluctuations, ensuring data integrity.
MRAM, a newer technology, uses magnetic tunnel junctions (MTJs) composed of thin magnetic layers to store data. MRAM offers several advantages over traditional memory technologies, including non-volatility, high speed, and low power consumption. Emerging MRAM technologies are exploring novel magnetic materials with enhanced properties for even greater storage density and performance.
## FAQ: Frequently Asked Questions About Magnetic Materials
Here are some frequently asked questions to further clarify the topic of magnetic materials:
**How do I choose the right magnetic material for my application?**
The selection process depends heavily on the specific requirements of your application. Consider factors such as the required magnetic strength, operating temperature, environmental conditions, cost, and size constraints.
**What are some common applications of hard magnetic materials?**
Hard magnets are used in a wide range of applications, including electric motors, generators, loudspeakers, magnetic separators, and holding devices.
**What are some common applications of soft magnetic materials?**
Soft magnets find use in transformer cores, inductors, electromagnetic shielding, and magnetic sensors.
**What is the difference between ferromagnetism and paramagnetism?**
Ferromagnetic materials exhibit strong, long-range ordering of atomic magnetic moments, leading to spontaneous magnetization. Paramagnetic materials have randomly oriented atomic moments that align partially with an external magnetic field, resulting in weak magnetization.
**What are the key limitations of using magnetic materials at high temperatures?**
High temperatures can lead to demagnetization due to increased thermal energy disrupting the alignment of magnetic moments. Selecting materials with high Curie temperatures is crucial for high-temperature applications.
**Are there any health or safety concerns associated with magnetic materials?**
While generally safe, strong magnetic fields can interfere with implanted medical devices like pacemakers. Proper handling and shielding are necessary to minimize potential risks, especially in industrial settings.
## Conclusion: Key Takeaways on Magnetic Material Comparison
Choosing the right magnetic material involves careful consideration of various factors, including coercivity, permeability, saturation magnetization, and Curie temperature. By understanding these properties, you can select the material that best suits your specific application.
Here’s a recap of the vital points we covered:
* **Coercivity:** Dictates resistance to demagnetization (hard vs. soft magnets).
* **Permeability:** Measures the ease of magnetic field formation within the material.
* **Saturation Magnetization:** Represents the maximum magnetic strength a material can achieve.
* **Curie Temperature:** Defines the point where a material loses its ferromagnetic properties.
* **Material Selection:** Alnico, Ferrite, NdFeB, SmCo, Silicon Steel, Mu-Metal, and amorphous metals each have unique strengths and weaknesses.
* **Temperature Effects:** High temperatures can degrade magnetic performance.
By carefully weighing these factors, you can confidently choose the best magnetic material for your project, optimizing performance and ensuring long-term reliability.
Compare and Contrast: A List of Magnetic Materials by Coercivity, Permeability, etc.
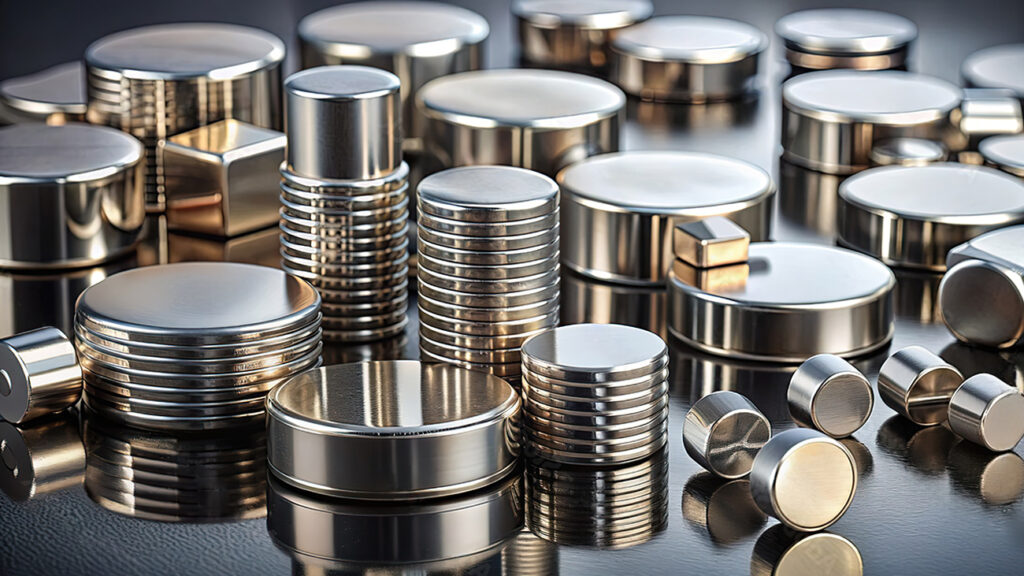