# Unveiling the Apex: Exploring the Most Powerful Magnet in the World and Its Astonishing Uses
Magnets, those seemingly simple devices that effortlessly attract certain metals, have come a long way from the humble lodestone. Today, we’re diving deep into the realm of extreme magnetism, exploring the mind-boggling power of the world’s strongest magnet, and uncovering its fascinating applications. This article will take you on a journey from the basics of magnetism to the forefront of scientific innovation, showcasing how this incredible technology is shaping our future. So, buckle up and prepare to be amazed!
## What Makes a Magnet “Powerful” and How Do We Measure It?
Before we can appreciate the sheer force of the strongest magnet, it’s crucial to understand what makes a magnet powerful in the first place. Magnetism, at its core, arises from the movement of electric charges. In most materials, these charges are randomly oriented, canceling out any overall magnetic effect. However, in certain materials like iron, nickel, and cobalt, the electron spins tend to align, creating microscopic magnetic domains. The more aligned these domains are, the stronger the magnet becomes.
The strength of a magnetic field is typically measured in **Tesla (T)**. One Tesla is a relatively strong magnetic field; for comparison, the Earth’s magnetic field is only about 0.00005 Tesla. Refrigerator magnets are about 0.01 Tesla. A powerful MRI machine might generate a field of 3 Tesla. Now, let’s think about those values when we talk about the world’s strongest magnet!
**Understanding Magnetic Field Strength:**
| Magnet Type | Approximate Field Strength (Tesla) |
|————————–|————————————|
| Earth’s Magnetic Field | 0.00005 |
| Refrigerator Magnet | 0.01 |
| MRI Machine | 3 |
| World’s Strongest Magnet| 45+ |
## Which Magnet Currently Holds the Title of “Most Powerful Magnet in the World?”
Currently, the title of “strongest magnet in the world” isn’t held by a single, static device. It’s a bit of a moving target! But, scientists at the National High Magnetic Field Laboratory (MagLab) in Tallahassee, Florida, have consistently been pushing the boundaries of magnet technology. They’ve created a variety of record-breaking magnets, but often, they are specialized for specific research purposes.
The world’s most powerful *resistive* magnet (a magnet that uses electricity to generate a magnetic field) is also at MagLab, achieving a sustained field of **45.5 Tesla**. This is an incredibly impressive feat of engineering. Superconducting magnets also exist, but they often face challenges in achieving such high sustained fields. The strongest pulsed magnet, using capacitors to release energy in short bursts, goes even higher (into the hundreds of Tesla), but the field is only maintained for milliseconds.
* **Key Fact:** The 45.5 Tesla resistive magnet at MagLab is considered the world’s strongest currently operational sustained-field magnet.
## What Materials Are Used to Construct Such Powerful Magnets?
Building a magnet capable of producing such intense magnetic fields requires a combination of advanced materials and innovative designs. They aren’t just simple iron bars! The materials used in these magnets include:
* **Niobium-Tin (Nb3Sn):** A superconducting material that can carry extremely high currents without resistance, crucial for producing strong magnetic fields efficiently. The current density is critical to this ability.
* **Niobium-Titanium (NbTi):** Another superconducting material, often used in combination with Nb3Sn for added strength and flexibility.
* **Copper Alloys:** High-strength copper alloys are used in resistive magnets to withstand the immense forces generated by the electric currents. These alloys often incorporate elements like beryllium or chromium to enhance their mechanical properties and electrical conductivity.
* **High-Strength Steel:** Surrounding components are crafted using high strength steel to withstand tremendous forces pulling the coils apart.
The combination of these materials and sophisticated winding techniques allows researchers to create magnets that can withstand extreme conditions and generate record-breaking magnetic fields.
## How Do Superconducting Magnets Differ from Resistive Magnets?
Superconducting magnets and resistive magnets are the two primary types used to generate strong magnetic fields, but they operate on fundamentally different principles:
* **Superconducting Magnets:** These magnets utilize materials that exhibit superconductivity at extremely low temperatures (typically near absolute zero). Superconductors offer zero electrical resistance, allowing for extremely high currents to be passed through the coils *without any energy loss*. This leads to much stronger and more stable magnetic fields with significantly lower energy consumption. However, they require complex and expensive cooling systems (often using liquid helium). They are generally used for sustained field applications in MRI machines and scientific research.
* **Resistive Magnets:** These magnets use conventional conductors, like copper, that offer resistance to the flow of electricity. This resistance generates heat, requiring significant cooling systems to prevent the magnet from overheating. They consume large amounts of electrical power and typically produce lower magnetic fields than superconducting magnets *for a given size and power consumption*. The advantage of resistive magnets is their ease of operation and robustness. They are commonly used in industrial applications and research settings where extremely high fields are needed for short durations.
* **Think of it this way:** Superconducting magnets are like hybrid cars – more efficient but requiring more complex infrastructure. Resistive magnets are like gas guzzlers – powerful but less efficient.
## What Are the Key Applications of the World’s Most Powerful Magnets?
These cutting-edge magnets aren’t just for breaking records; they have a wide range of incredibly important applications across different fields:
1. **Materials Science:** Studying the behavior of materials under extreme magnetic fields, leading to the discovery of new materials with unique properties. For example, high magnetic fields can alter the electronic structure of materials, revealing novel quantum phenomena like superconductivity and topological phases.
2. **Chemistry:** Probing chemical reactions and molecular structures in detail. Nuclear Magnetic Resonance (NMR) spectroscopy relies on strong magnetic fields to analyze the structure and dynamics of molecules. Higher magnetic fields offer greater resolution and sensitivity in NMR experiments.
3. **Medicine:** Advancing medical imaging techniques like Magnetic Resonance Imaging (MRI), providing higher resolution images for better diagnosis. Stronger magnetic fields improve image quality, allowing doctors to visualize finer details and detect subtle abnormalities.
4. **Fusion Energy Research:** Confining plasmas in fusion reactors, a crucial step towards developing sustainable energy sources (more on this below). Magnetically confined fusion is a critical avenue for achieving controlled thermonuclear reactions and potentially limitless clean energy.
5. **Particle Physics:** Guiding and manipulating particle beams in accelerators, enabling scientists to probe the fundamental building blocks of matter. High-energy particle colliders, such as the Large Hadron Collider (LHC), rely on powerful magnets to steer particles travelling at near-light speed around the accelerator ring.
6. **Spintronics:** Developing new electronic devices that utilize the spin of electrons, potentially leading to faster and more energy-efficient computers. Strong magnetic fields are essential for controlling and manipulating electron spin in spintronic materials and devices.
## What Role Do These Magnets Play in Fusion Energy Research?
Fusion energy, the process that powers the sun, holds immense promise as a clean and virtually limitless energy source. Harnessing this power on Earth requires confining extremely hot plasma (a superheated state of matter) within a magnetic field strong enough to prevent it from touching the reactor walls. If the plasma touches the walls, it cools down and the fusion reaction stops.
Tokamaks, one of the most promising designs for fusion reactors, use powerful magnets to create a “magnetic bottle” that confines the plasma. The stronger the magnetic field, the better the plasma confinement and the higher the efficiency of the fusion reaction. Scientists are constantly striving to create even stronger magnets to improve plasma confinement and achieve sustainable fusion energy. The increased magnetic field strength translates to higher plasma density, and, therefore, higher fusion power.
* **Key Concept:** Stronger magnets = better plasma confinement = more efficient fusion energy production.
## How Does the Use of Strong Magnets Enhance MRI Technology?
Magnetic Resonance Imaging (MRI) is a revolutionary medical imaging technique that uses strong magnetic fields and radio waves to create detailed images of the organs and tissues inside the body. Stronger magnetic fields in MRI machines offer several advantages:
* **Improved Image Resolution:** Higher field strengths allow for better spatial resolution, enabling doctors to visualize smaller structures and detect subtle abnormalities that might be missed with lower field strengths.
* **Enhanced Signal-to-Noise Ratio:** Stronger magnetic fields increase the signal-to-noise ratio, resulting in clearer and more detailed images.
* **Faster Scan Times:** With stronger magnets, it is possible to acquire images more quickly. This reduces the time patients need to spend inside the machine.
* **Specialized Imaging Techniques:** Stronger magnetic fields enable advanced MRI techniques, such as functional MRI (fMRI), which measures brain activity by detecting changes in blood flow.
While standard MRI machines typically operate at 1.5-3 Tesla, research-grade MRI systems can use magnets as strong as 7 Tesla or even higher, providing unparalleled image quality. These high-field MRI systems offer enormous potential for advancing medical research and improving patient care.
## What Challenges Are Involved in Creating and Maintaining Such Powerful Magnets?
Building and maintaining the world’s most powerful magnets is a formidable engineering challenge. Here are some key hurdles:
* **Immense Forces:** The magnetic forces generated by these magnets are enormous. The coils must be designed to withstand these forces, preventing them from deforming or breaking apart.
* **Extreme Cooling:** Resistive magnets generate a significant amount of heat due to the electrical resistance of the conductors. Cooling systems must be designed to dissipate this heat and prevent the magnet from overheating. Superconducting magnets require cryogenic cooling to maintain their superconducting state.
* **Material Limitations:** Finding materials that can withstand the extreme forces and temperatures required for these magnets is a constant challenge. Researchers are continuously investigating new materials and fabrication techniques to improve magnet performance.
* **High Manufacturing Costs:** Fabricating these magnets requires specialized equipment and expertise, resulting in high manufacturing costs.
* **Quench Protection:** In superconducting magnets, a “quench” occurs when the superconductor suddenly loses its superconducting properties. This can lead to a rapid release of energy. Safety systems become vital to protect the coil.
Overcoming these challenges requires a multidisciplinary approach involving materials science, electrical engineering, mechanical engineering, and physics.
## What Future Innovations Can We Expect in Magnet Technology?
The field of magnet technology is constantly evolving, with researchers pushing the boundaries of what’s possible. Some exciting future innovations include:
* **Higher Temperature Superconductors:** Developing superconducting materials that can operate at higher temperatures, reducing the need for expensive cryogenics. This would make superconducting magnets more practical and widely accessible.
* **New Magnet Designs:** Exploring novel magnet designs that can achieve stronger magnetic fields with less material and lower energy consumption.
* **Advanced Manufacturing Techniques:** Utilizing advanced manufacturing techniques, such as additive manufacturing (3D printing), to create complex magnet geometries with improved performance.
* **Compact Fusion Reactors:** Developing smaller, more efficient fusion reactors that utilize high-field magnets to confine the plasma. This could lead to the development of practical and affordable fusion power plants.
* **Quantum Computing:** Exploring the use of strong magnetic fields in quantum computing, potentially enabling the development of more powerful and versatile quantum computers.
These advances promise to revolutionize various fields, including medicine, energy, and materials science. The continuous pursuit of stronger and more efficient magnets will undoubtedly lead to groundbreaking discoveries and transformative technologies.
## FAQ Section: Answering Your Questions about Powerful Magnets
Here are some frequently asked questions about the world’s most powerful magnets:
**永久磁石と電磁石の違いは何ですか?
Permanent magnets, like those on your refrigerator, have their magnetic field inherent in the material itself. These are typically made from materials that have aligned atomic magnetic moments. Electromagnets, on the other hand, generate a magnetic field only when electricity runs through a coil of wire. Turning off the electricity stops the magnetic field. The world’s most powerful magnets are almost exclusively electromagnets, since it is possible to control electricity flow and amplify the magnetic field far beyond what a permanent magnet can generate.
**Are there any dangers associated with working near extremely powerful magnets?**
Yes, there are significant risks. These magnets can attract ferromagnetic materials (like iron and steel) with tremendous force. Small metal objects, such as tools or even watches, can become dangerous projectiles. High magnetic fields can also interfere with medical implants like pacemakers. Stringent safety protocols must be in place to protect personnel and equipment. Magnetic fields can also scramble data on magnetic storage devices like hard drives and credit cards.
**How are these magnets cooled to prevent them from overheating or losing their superconducting properties?**
Resistive magnets are typically cooled using water or liquid nitrogen to dissipate the heat generated by the electrical resistance. Superconducting magnets are cooled using liquid helium, which is maintained at extremely low temperatures (around -269 degrees Celsius). This cryogenic cooling system is essential to maintain the superconducting state.
**Why can’t we just make all magnets stronger and stronger?**
There are fundamental limitations. As the magnetic field strength increases, the forces acting on the magnet’s structure become immense, challenging the material’s strength. Achieving even incrementally higher fields requires significant advancements in materials science, engineering designs, and cooling technologies. The cost and complexity also escalate rapidly with increasing field strength.
**Where can I learn more about magnet technology and research?**
The National High Magnetic Field Laboratory (MagLab) is an excellent resource for learning about magnet technology and research. Websites of universities and research institutions involved in magnet development often publish related articles and research papers. Scientific journals such as *Nature*, *Science*, and *Physical Review Letters* also feature cutting-edge research in this area.
## Conclusion: The Amazing World of Powerful Magnets – Key Takeaways
* The world’s most powerful sustained magnetic field is currently generated by a 45.5 Tesla resistive magnet at the National High Magnetic Field Laboratory.
* These powerful magnets rely on advanced materials, like niobium-tin and copper alloys, and innovative designs.
* Superconducting magnets offer greater efficiency but require cryogenic cooling, while resistive magnets are more robust but consume more energy.
* Key applications include materials science, chemistry, medicine (MRI), fusion energy research, and particle physics
* They play a crucial role in confining plasma in fusion reactors and improving medical imaging in MRI machines.
* Building and maintaining these magnets involves overcoming immense forces, extreme cooling requirements, and material limitations.
* Future innovations promise even stronger magnets, higher temperature superconductors, and compact fusion reactors.
The pursuit of stronger magnets continues to drive scientific discovery and technological innovation, with the potential to transform our world in profound ways.
The Most Powerful Magnet in the World (and What It’s Used For)
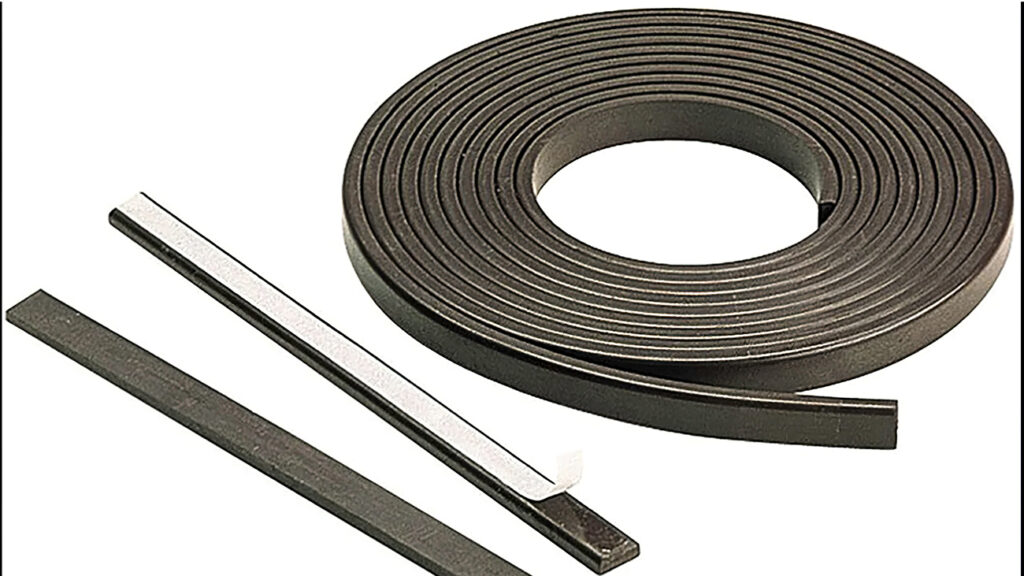