# Revolutionizing Technology: A Look at Advances in Novel Magnetic Materials Compositions
In today’s rapidly evolving technological landscape, magnetic materials play a crucial role in countless applications, from data storage and energy conversion to medical imaging and advanced sensors. This article dives deep into the exciting world of **advances in magnetic materials: a list of novel compositions,** exploring the latest breakthroughs that promise to reshape industries and drive innovation. We’ll uncover the secrets behind these cutting-edge materials and understand why they are essential for the future of technology. Get ready to explore the fascinating world of magnetism!
## 1. What are the Key Properties Driving Research in New **Magnetic Material Compositions**?
The quest for novel magnetic materials is driven by the desire to enhance several key properties:
* **High Saturation Magnetization (Ms):** This determines the maximum magnetic moment a material can achieve, crucial for applications like high-density data storage. A material with a higher Ms can store data more efficiently. Think of it like filling a bucket with sand – a bigger bucket (higher Ms) can hold more sand (data).
* **High Curie Temperature (Tc):** This is the temperature above which a material loses its ferromagnetic properties. A higher Tc ensures that the material functions effectively at higher operating temperatures. Imagine a magnet that works perfectly until it gets a little warm, and then it stops being a magnet; that’s what we want to avoid!
* **High Magnetic Anisotropy (Ku):** This describes the material’s preference for magnetization along a specific direction, vital for maintaining data stability in magnetic recording. We want our data to stay put, and high anisotropy helps prevent it from accidentally flipping.
* **Low Magnetic Losses:** Minimizing energy dissipation during magnetization and demagnetization cycles is essential for energy-efficient applications. Reducing loss is like making a more efficient engine; it wastes less energy.
Researchers are constantly tweaking the chemical composition and structure of materials to achieve these desired properties. It’s like a chef experimenting with ingredients to create the perfect dish! Understanding these properties is key to unlocking the full potential of magnetic materials.
## 2. How are Rare-Earth Free **Permanent Magnetic Materials** Revolutionizing Industries?
Rare-earth elements, such as neodymium and dysprosium, are essential components in high-performance permanent magnets. However, their limited availability and geopolitical concerns have spurred research into rare-earth-free alternatives.
* **Manganese Bismuth (MnBi):** MnBi based magnets exhibit excellent high temperature performance, with a high Curie temperature and potentially high coercivity.
* **Iron Nitrides (FeN):** FeN compounds can achieve high saturation magnetization, making them promising candidates for cheaper, more sustainable magnets. These are still under development and require further refinement.
* **Iron-Cobalt Alloys (FeCo):** By carefully controlling the composition and processing, FeCo alloys can exhibit competitive magnetic properties without relying on rare earths.
Replacing rare earths with more abundant elements is a major focus. This not only reduces cost but also lowers environmental impact, making these new magnets much more sustainable. It’s a win-win for technology and the planet!
## 3. What are the Advantages of **Nanocrystalline Soft Magnetic Materials**?
Nanocrystalline soft magnetic materials, composed of ultra-fine grains (typically less than 100 nm), offer unique advantages:
* **High Permeability:** They can easily be magnetized and demagnetized, making them ideal for applications in transformers, inductors, and sensors. High permeability means the material can concentrate magnetic fields effectively.
* **Low Coercivity:** They require very little magnetic field to change their magnetization state, minimizing energy losses. Low coercivity means less energy is needed to switch the magnet on and off.
* **High Resistivity:** This reduces eddy current losses, further improving energy efficiency, particularly at high frequencies. High resistivity prevents unwanted electrical currents from flowing within the material, which can waste energy.
These materials are like incredibly flexible magnets – they respond quickly and efficiently to changes in magnetic fields. This makes them perfect for applications where speed and efficiency are paramount.
**Example:** Nanocrystalline alloys like Finemet (FeCuNbSiB) are widely used in transformer cores, leading to significant energy savings.
| Property | Finemet (FeCuNbSiB) | Traditional Silicon Steel |
|————–|———————-|—————————|
| Permeability | High | Moderate |
| Core Loss | Low | High |
## 4. How are **Heusler Alloys** Contributing to Spintronics?
Heusler alloys are intermetallic compounds with a wide range of functionalities, including ferromagnetism, half-metallicity, and shape memory effects. Their unique electronic structure makes them promising for spintronics applications.
* **Spin Polarization:** Some Heusler alloys exhibit nearly 100% spin polarization at the Fermi level, meaning that only electrons with a particular spin orientation contribute to the current. This is crucial for highly efficient spin injection and manipulation.
* **Giant Magnetoresistance (GMR):** Heusler alloys can be used in GMR devices, where a small change in magnetic field leads to a large change in electrical resistance. This effect is the basis for modern hard drive read heads.
* **Tunnel Magnetoresistance (TMR):** Similar to GMR, TMR relies on quantum mechanical tunneling of electrons through a thin insulating barrier. Heusler alloys can significantly enhance TMR ratios, boosting device performance.
Heusler alloys are like the superheroes of spintronics – they have special abilities that allow them to control the spin of electrons in amazing ways! This could lead to a new generation of faster, more energy-efficient electronic devices.
## 5. What Role do **Ferrites** Play in High-Frequency Applications?
Ferrites are ceramic materials composed of iron oxide and other metal oxides. They are widely used in high-frequency applications due to their:
* **High Resistivity:** This minimizes eddy current losses at high frequencies, allowing for efficient performance in inductors, transformers, and microwave devices.
* **Good Magnetic Permeability:** They can effectively concentrate magnetic fields at specific frequencies, enhancing device performance.
* **Low Cost:** They are relatively inexpensive to produce, making them a cost-effective option for many applications.
Think of ferrites as the reliable workhorses of high-frequency electronics. They might not be as flashy as some other materials, but they get the job done consistently and efficiently.
**Example:** Ferrites are commonly used in inductors and transformers in switched-mode power supplies used in cell phone chargers and laptop power adapters.
## 6. Can **Amorphous Magnetic Alloys** Enhance Power Electronics?
Amorphous magnetic alloys, lacking a long-range crystalline order, exhibit unique properties:
* **High Permeability:** They can be easily magnetized even under weak field which enable higher efficiencies.
* **Low Core Loss:** Due to the absence of crystalline defects and magnetocrystalline anisotropy, amorphous alloys exhibit low hysteresis losses, leading to improved energy efficiency.
* **High Saturation Induction:** This allows for smaller and lighter magnetic components.
These properties make them attractive for applications in power electronics, where efficiency and size are critical.
These alloys can be thought of as magnets with a free-flowing structure in their elements, which allows them to perform consistently, efficiently, and under much less resistance.
**Case Study:** Hitachi Metglas amorphous alloys are used in distribution transformers, resulting in significant energy savings for utility companies.
## 7. Are **Multiferroic Materials** the Future of Memory Storage?
Multiferroic materials exhibit two or more ferroic orders (ferroelectricity, ferromagnetism, ferroelasticity) simultaneously. This opens up the possibility of controlling magnetism with electric fields and vice versa, leading to new device concepts.
* **Electric Field Control of Magnetism:** This allows for energy-efficient manipulation of magnetic states, which could revolutionize memory storage.
* **Non-Volatile Memory:** Multiferroic materials can be used to create non-volatile memory devices that retain data even when power is off.
* **New Sensing Technologies:** The coupling between electric and magnetic properties can be exploited to create highly sensitive sensors.
Multiferroics are like magnets that can be controlled with electricity – imagine the possibilities! This could lead to a new era of ultra-low-power electronic devices.
## 8. How are **Exchange Spring Magnets** Boosting Data Storage Density?
Exchange spring magnets consist of a “hard” magnetic layer coupled to a “soft” magnetic layer. This combination allows for achieving high coercivity and high saturation magnetization simultaneously.
* **Enhanced Coercivity:** The hard layer provides stability, preventing data from being erased by external magnetic fields.
* **High Saturation Magnetization:** The soft layer contributes to a high overall magnetic moment, allowing for increased data storage density.
* **Improved Thermal Stability:** Exchange spring magnets can maintain their magnetic properties at elevated temperatures, crucial for reliable data storage.
Imagine a magnet with a tough core and a powerful outer layer – that’s an exchange spring magnet! This design allows for storing more data while keeping it safe and secure.
## 9. What is the Potential of **Molecular Magnets** in Quantum Computing?
Molecular magnets, also known as single-molecule magnets (SMMs), are individual molecules that exhibit magnetic behavior.
* **Quantum Coherence:** SMMs can maintain quantum coherence for relatively long periods, making them promising candidates for building blocks of quantum computers.
* **Tunable Magnetic Properties:** The magnetic properties of SMMs can be tailored by modifying their chemical structure.
* **High Density:** SMMs can be packed together at very high densities, potentially leading to miniaturized quantum devices.
These magnets, working as single components, act as potential processors of quantum computers and are a potential future in tech memory and computing.
Molecular magnets are like tiny, customizable magnets with quantum superpowers – they could revolutionize the way we process information!
## 10. Where is Research Headed for **Metamaterials** with Novel Magnetic Properties?
Metamaterials are artificially engineered materials with properties not found in nature. By carefully designing their structure, we can create materials with unusual magnetic behavior.
* **Negative Permeability:** Metamaterials can exhibit negative magnetic permeability, allowing for manipulation of magnetic fields in novel ways.
* **Cloaking Devices:** Metamaterials can be used to create cloaking devices that render objects invisible to magnetic fields.
* **Perfect Lenses:** Metamaterials can be designed to focus magnetic fields with unprecedented precision, enabling new imaging and sensing technologies.
Metamaterials are like magic cloaks for magnets – they can bend and shape magnetic fields in amazing ways! This could lead to new applications in everything from invisibility to advanced medical imaging.
## 자주 묻는 질문(FAQ)
* What is saturation magnetization, and why is it important?
Saturation magnetization refers to the maximum magnetic moment a material can achieve. A higher Ms is crucial for applications like high-density data storage because it allows for storing more information in a smaller space. It’s like having a bigger memory stick for your computer.
* Why is there so much research into rare-earth-free magnets?
Rare-earth elements are essential for high-performance magnets but are becoming harder to find. Additionally geopolitical concerns and environmental impact considerations are driving research into more sustainable and widely available alternatives.
* What are the advantages of using amorphous alloys in power electronics?
Amorphous alloys offer high permeability, low core loss, and high saturation induction, making them more efficient and compact.
* How could multiferroic materials revolutionize memory storage?
Multiferroic materials can be controlled with electric fields, allowing for energy-efficient manipulation of magnetic states and enabling non-volatile memory devices that retain data even when power is off.
* What are exchange spring magnets, and how do they enhance data storage?
Exchange spring magnets combine a “hard” magnetic layer with a “soft” magnetic layer to achieve high coercivity and saturation magnetization, leading to improved data storage density and thermal stability.
* Where can I learn more about the latest advances in magnetic materials?
You can find up-to-date, peer reviewed research on magnetic material from resources such as scientific journals (Nature, Science, Advanced Materials), industry conferences (Intermag, MMM), and reports published by research institutions.
## 결론: 주요 요점
* The quest for new **magnetic materials** is driven by the need for higher performance, sustainability, and energy efficiency.
* **Rare-earth-free magnets** are a promising alternative to traditional magnets, addressing concerns about resource availability and environmental impact.
* **Nanocrystalline alloys** and **Amorphous metal alloys** continue to improve efficiency in motors, transformers, and sensing applications
* **Heusler alloys** and will be crucial to future device innovations via spintronics.
* **Multiferroic materials** offer the potential for revolutionary memory storage technologies.
* Advances in **metamaterials** promise to unlock new possibilities for manipulating magnetic fields, from cloaking to advanced imaging.
* **Molecular magnets** may be the foundation for future innovations in quantum computing.
The future of magnetic materials is bright, with ongoing research continuously pushing the boundaries of what’s possible. These advancements promise to shape the world around us, improving everything from the energy we use to the way we store and process information.
Advances in Magnetic Materials: A List of Novel Compositions
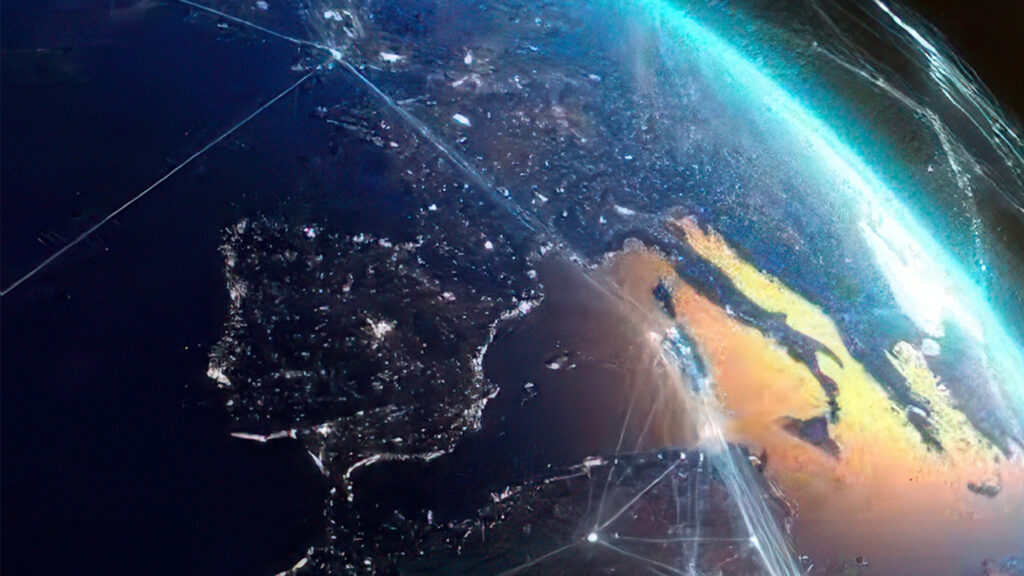