# High-Powered Magnets: Levitation, Fusion Energy, & The Future of Technology
High-powered magnets are no longer just for novelty toys. They’re rapidly evolving into crucial components of groundbreaking technologies, from revolutionary transportation systems using magnetic levitation (Maglev) to the pursuit of clean energy through nuclear fusion. This article will explore the fascinating world of high-powered magnet technology, covering levitation, fusion energy, magnetic resonance imaging (MRI), and other exciting applications. I’ll break down the science in an easy-to-understand way and explain why these advancements are vital to shaping our future.
## What Exactly are High-Powered Magnets?
High-powered magnets, often made from rare-earth elements like neodymium and samarium, possess significantly stronger magnetic fields than conventional magnets. The strength of a magnet is generally measured in Teslas (T). While a refrigerator magnet might produce a field of around 0.001 T, high-powered magnets can generate fields of several Teslas, allowing for forces strong enough to lift heavy objects or confine plasma in fusion reactors.
These powerful magnetic fields are created by aligning the magnetic moments of the atoms within the material. Advanced manufacturing techniques enable scientists to create magnets with precisely controlled shapes and magnetic properties. This precision is essential for their use in complex technological systems.
## How Does Magnetic Levitation (Maglev) Work, and What are its Potential Benefits?
Magnetic levitation, or Maglev, is a transportation technology that uses powerful magnets to suspend, guide, and propel vehicles without physical contact with the track. This eliminates friction, allowing trains to reach speeds of up to 375 mph or even higher.
There are two main types of Maglev systems:
* **Electromagnetic Suspension (EMS):** EMS systems use electromagnets on the train that are attracted to a steel rail. The attraction lifts the train, and sensors constantly adjust the magnetic field to maintain a consistent gap.
* **Electrodynamic Suspension (EDS):** EDS systems use superconducting magnets on the train that interact with a conductive guideway. The movement of the magnets induces currents in the guideway, creating an opposing magnetic field that repels the train upward.
The benefits of Maglev are numerous: faster travel times, reduced noise pollution, lower maintenance costs (due to less wear and tear), and increased energy efficiency compared to traditional rail systems. While the initial investment can be high, the long-term operational advantages make Maglev a promising transportation solution.
## Can High-Powered Magnets Help Us Achieve Clean Fusion Energy?
Nuclear fusion, the process that powers the sun, holds the potential for virtually limitless clean energy. However, achieving sustained fusion on Earth is a monumental challenge. One of the most promising approaches involves using high-powered magnets to confine a plasma of deuterium and tritium (hydrogen isotopes) at extremely high temperatures (over 100 million degrees Celsius).
These magnets, arranged in configurations like tokamaks or stellarators, create a powerful magnetic field that prevents the plasma from touching the walls of the reactor. If the plasma were to touch the walls, it would cool down, stopping the fusion reaction. The magnetic fields must be extremely strong and carefully shaped to maintain plasma stability.
Here’s a simple table summarizing the advantages of fusion energy:
| Advantage | Description |
| —————— | ——————————————————————————- |
| Abundant Fuel | Deuterium can be extracted from seawater, and tritium can be produced from lithium. |
| Clean Energy | Fusion produces no greenhouse gases or long-lived radioactive waste. |
| Inherently Safe | Fusion reactors are designed to shut down automatically in case of a problem. |
| High Energy Output | A small amount of fuel can produce a large amount of energy. |
ITER (International Thermonuclear Experimental Reactor) in France is a major international collaboration aimed at demonstrating the feasibility of fusion power. It relies on some of the most powerful magnets ever built.
## How are High-Powered Magnets Used in Medical Imaging (MRI)?
Magnetic Resonance Imaging (MRI) is a powerful medical imaging technique that uses strong magnetic fields and radio waves to create detailed images of the organs and tissues inside the body. High-powered magnets are essential for MRI because they produce the strong, uniform magnetic field required for high-resolution imaging.
The strong magnetic field aligns the protons in the body. Radio waves are then emitted, which temporarily disrupt this alignment. As the protons realign themselves, they emit signals that are detected by the MRI scanner. These signals are then processed by a computer to create an image. Higher field strength results in better image quality and faster scan times. MRI is invaluable for diagnosing a wide range of conditions, from brain tumors to torn ligaments.
Statistics About MRI:
* Approximately 40 million MRI scans are performed each year in the U.S.
* The average MRI machine uses a magnetic field strength of 1.5 to 3 Tesla.
* Research is ongoing to develop even higher field MRI scanners for improved imaging.
## Beyond Levitation and Fusion: What Other Applications Exist for High-Powered Magnets?
High-powered magnets have applications extending far beyond levitation and fusion. I have found some truly remarkable uses:
* **Particle Accelerators:** Used to steer and focus beams of particles in scientific research.
* **Electric Motors and Generators:** Improve efficiency and power density in electric vehicles and wind turbines.
* **Magnetic Separation:** Used to separate materials in mining, recycling, and wastewater treatment.
* **Data Storage:** Enabling higher storage densities in hard drives.
* **High-Speed Trains** As described above, they are essential to maglev, which is a high-speed train.
## What Challenges Faced in Developing and Using High-Powered Magnets?
Despite their enormous potential, I have found that there are significant challenges associated with creating and using high-powered magnets:
* **Cost:** Rare-earth elements used in high-powered magnets are expensive and can be subject to supply chain disruptions.
* **Heat Management:** Superconducting magnets require extremely low temperatures (close to absolute zero), which requires complex and energy-intensive cooling systems.
* **Magnetic Forces:** High-powered magnets generate enormous forces that can be difficult to contain and control.
* **Safety:** Strong magnetic fields can interfere with electronic devices and pose a risk to people with pacemakers or metal implants.
## What are Superconducting Magnets, and How Do They Improve Performance?
Superconducting magnets are a special type of electromagnet that use superconducting materials to generate extremely strong magnetic fields. Superconducting materials have zero electrical resistance when cooled below a critical temperature. This means that a current can flow through them indefinitely without losing energy.
This zero resistance allows superconducting magnets to achieve much higher magnetic field strengths than conventional electromagnets, which are limited by the amount of current they can carry before overheating. They are therefore essential in applications such as MRI and nuclear fusion.
Here is a numbered list covering how to make a simple electromagnet:
1. Gather your materials: You will need an iron nail, insulated copper wire, a battery (1.5V to 9V), and some small metal objects (like paper clips or thumbtacks).
2. Wrap the wire around the nail: Starting a few inches from one end, tightly wrap the insulated copper wire around the iron nail. Make as many turns as possible, keeping the coils close together. Leave a few inches of wire free at each end of the nail.
3. Prepare the wire ends: Carefully strip about an inch of insulation from both ends of the copper wire. This is important because the electricity needs direct contact with the metal to flow.
4. Connect the wire to the battery: Attach one end of the stripped wire to the positive (+) terminal of the battery and the other end to the negative (-) terminal. The current will now flow through the wire, turning the nail into an electromagnet.
5. Test your electromagnet: Bring the nail close to the small metal objects you gathered. The electromagnet should attract and pick them up.
6. Disconnect when done: When you are finished experimenting, disconnect the wire from the battery. This prevents the battery from draining and the wire from overheating.
Superconducting wires are a more complex technology than copper wire, but the principle of applying current and creating a magnetic field is the same.
## How is Materials Science Advancing High-Powered Magnet Technology?
Materials science plays a crucial role in advancing high-powered magnet technology. Researchers are constantly developing new materials with improved magnetic properties, higher critical temperatures for superconductivity, and greater resistance to degradation under extreme conditions.
Recent advances include the development of:
* High-temperature superconductors that can operate at higher temperatures, reducing the cost and complexity of cooling systems.
* Nanocomposite magnets with enhanced magnetic properties and mechanical strength.
* Rare-earth-free magnets that reduce reliance on scarce and environmentally problematic materials.
## What are the Economic and Societal Implications of Widespread Adoption of These Technologies?
If the described magnet technologies reach widespread adoption, they can have large positive effects on economies and society, especially those related to carbon emissions.
* **Transportation:** Faster and more efficient Maglev trains could revolutionize long-distance travel and reduce reliance on air travel.
* **Energy:** Fusion energy could provide a virtually limitless source of clean energy, mitigating climate change and ensuring energy security.
* **Healthcare:** Improved MRI technology could lead to earlier and more accurate diagnoses of diseases.
* **Manufacturing:** Magnetic separation technologies could improve efficiency in mining, recycling, and wastewater treatment.
However, it is also important to consider the potential economic and social challenges:
* **Job displacement:** Automation resulting from advanced magnetic technologies could lead to job losses in certain sectors.
* **Accessibility:** Ensuring that the benefits of these technologies are distributed equitably and are not limited to wealthy nations.
* **Environmental concerns:** Addressing the environmental impacts of mining rare-earth elements and manufacturing high-powered magnets.
## What Future Breakthroughs Can We Anticipate in the Realm of High-Powered Magnet Technology?
I anticipate that the future of high-powered magnet technology will be a blend of incremental optimization of what exists today and big breakthroughs on the horizon. Specifically, I think the following areas of research hold a ton of opportunity for future improvement and discoveries:
* **Higher-temperature Superconductors:** Room-temperature superconductors would revolutionize magnet technology, eliminating the need for expensive cooling systems.
* **Ultra-Strong Magnets:** Developing magnets with even higher field strengths would enable new applications in fusion energy, particle physics, and materials science.
* **Wireless Power Transfer** Using high-powered magnets to transfer electricity wirelessly over long distances.
## Frequently Asked Questions (FAQ)
Why are rare-earth elements used in many high-powered magnets?
Rare-earth elements, such as neodymium and samarium, have unique atomic structures that allow them to create very strong magnetic fields. This is because they have a large number of unpaired electrons, which all align in the same direction, creating a strong magnetic dipole.
How do superconducting magnets stay cold?
Superconducting magnets are cooled using liquid helium, which has an extremely low boiling point (around -269 degrees Celsius). The liquid helium is circulated through the magnet to keep the superconducting material below its critical temperature.
Are strong magnetic fields harmful to humans?
Strong magnetic fields can be harmful to humans in certain circumstances. They can interfere with electronic devices, such as pacemakers, and can cause metal objects to become projectiles. Therefore, it is important to follow safety precautions around high-powered magnets.
What is the difference between a permanent magnet and an electromagnet?
A permanent magnet creates its own magnetic field, while an electromagnet requires an electric current to generate a magnetic field. Permanent magnets retain their magnetism even when there is no external current, while electromagnets only produce a magnetic field when current is flowing.
How does Magnetic Resonance Imaging work?
MRI uses strong magnetic fields and radio waves to create detailed images of the organs and tissues inside the body. The strong magnetic field aligns the protons in the body. Radio waves are then emitted, which temporarily disrupt this alignment. As the protons realign themselves, they emit signals that are detected by the MRI scanner.
Can magnets really levitate trains?
Yes, magnetic levitation (Maglev) trains use powerful magnets to suspend, guide, and propel vehicles without physical contact with the track, eliminating friction.
## Conclusion
As I explored in this article, high-powered magnet technology is a dynamic field with the potential to transform multiple facets of our lives, from transportation and energy to healthcare and manufacturing. While the challenges are significant, the advances being made in materials science and engineering are paving the way for exciting breakthroughs.
Let’s recap the key takeaways:
* High-powered magnets are used in a wide range of applications, including Maglev trains, fusion reactors, and MRI scanners.
* Superconducting magnets can achieve much higher magnetic field strengths than conventional electromagnets.
* Materials science is playing a crucial role in developing new magnets with improved properties.
* The widespread adoption of these technologies could have significant economic and societal implications.
* Future breakthroughs could include room-temperature superconductors and ultra-strong magnets.
Levitation, Fusion, and More: High-Powered Magnet Technologies Shaping the Future
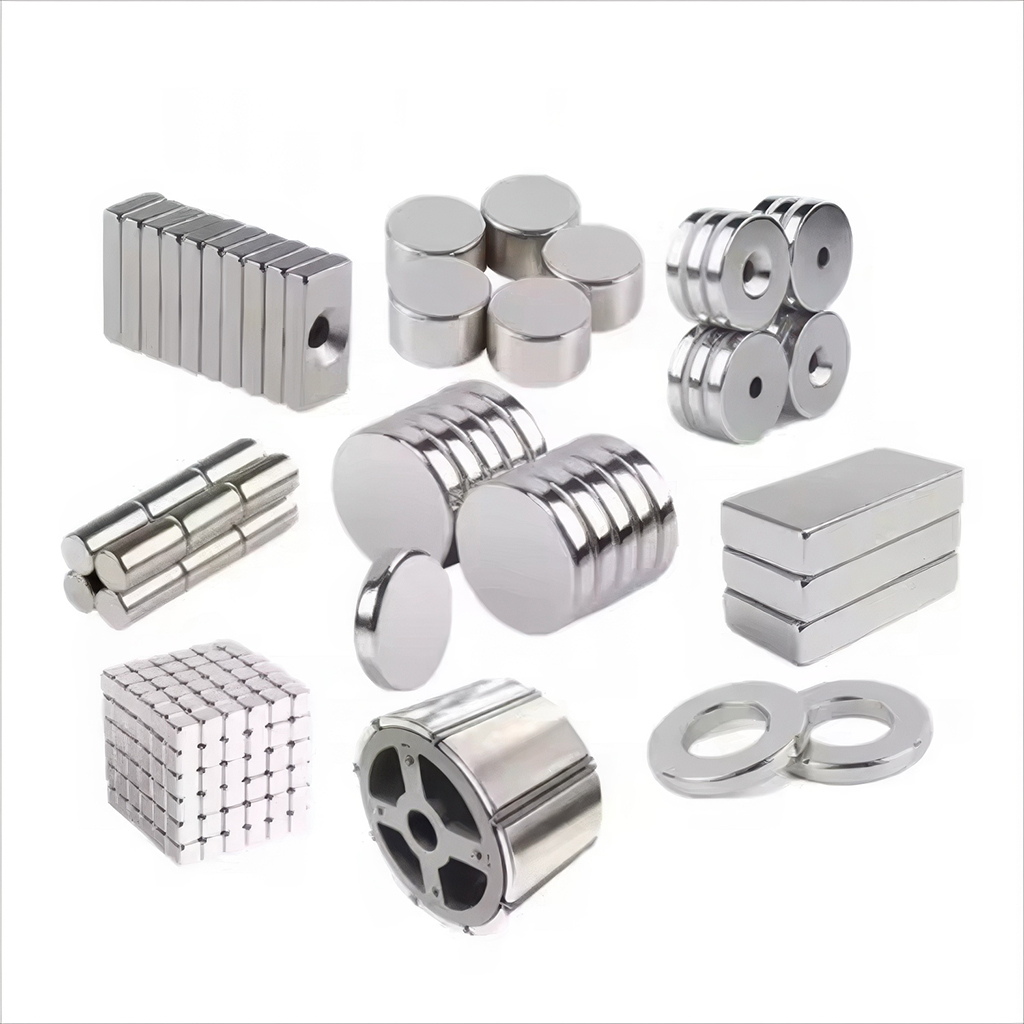